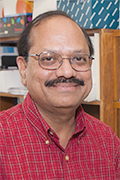
M. Mahmood Hussain, PhD, Lic. Med.
Professor
Department of Cell Biology
- MD
- PhD
- MS
- MPH
High plasma lipid and lipoprotein levels are a risk factor for atherosclerosis. Lowering plasma lipid levels is a national goal and new approaches to decrease lipid levels are required. Our long-term goal is to understand the molecular assembly of lipoproteins and to use this knowledge to lower plasma lipid levels. To achieve this, we are trying to understand specific issues related to the assembly and catabolism of lipoproteins. These studies have implications in cardiovascular and neurodegenerative disorders.
1. BIOSYNTHESIS OF CHYLOMICRONS
We would like to understand various molecular steps involved in the assembly and secretion of chylomicrons. Chylomicrons are lipoproteins assembled to transport dietary fat and fat-soluble vitamins. Understanding of chylomicron assembly has lagged far behind the knowledge of lipoprotein assembly by hepatocytes due to a lack of cell culture model. We have developed a cell culture model to study the assembly of intestinal lipoproteins. At present, the role of apoB and microsomal triglyceride transfer protein (MTP) in the assembly of chylomicrons is under investigation. In addition, we are studying the mechanism of assembly of individual lipid components (triglycerides, phospholipids, cholesterol, vitamin A, vitamin E etc.) into these particles. The knowledge of chylomicron assembly may help us to devise interventions that can inhibit lipid absorption and lower plasma cholesterol. Our working model is depicted below.
Figure 1
Biochemical signposts for different stages of chylomicron assembly
1. Primordial lipoproteins with preformed phospholipids: The nascent apoB molecule is shown to interact with the inner leaflet of the endoplasmic reticulum membrane. The site of apoB/membrane interactions is depicted to start concentrating neutral lipids. This process could be mediated by MTP. After substantial transfer of neutral lipids, the apoB may bud off as a small spherical particle. In this process, phospholipids present in the inner leaflet of the endoplasmic reticulum may form the outer layer of the primordial lipoproteins. Metabolic labeling experiments show that newly synthesized phospholipids are not targeted to the site of lipoprotein assembly and "preformed phospholipids" are used for the assembly of intestinal lipoproteins (1). Thus, it is proposed that incorporation/association of preformed phospholipids with nascent apoB can be used as a signpost for this step. 2. Lipid droplets with nascent triglycerides: It is hypothesized that under basal conditions small triglyceride-rich particles are formed independent of apoB. In the postprandial state, such as increased availability of oleic acid (OA), the size of these particles increases due to the incorporation of nascent triglycerides. The formation of larger lipid droplets is hypothesized to be especially sensitive to Pluronic L81. 3. Core expansion: This step may involve fusion of primordial lipoprotein particles with triglyceride-rich lipid droplets of various sizes to give rise to lipoprotein particles of different sizes. This step is crucial for the transport of large amounts of triglycerides. 4. Addition of retinyl esters. Retinyl esters are secreted only with chylomicrons and not with other lipoproteins such as VLDL, IDL and LDL (2). Thus, they may serve as signposts for the final stages of chylomicron assembly. At this time, the basis for the specificity of the incorporation of retinyl esters to chylomicrons is unknown. 5. Secretion. This step represents the secretion of nascent lipoproteins. The signals for the secretion of nascent lipoproteins are not known. Most likely, secretion of lipoproteins is a constitutive process. Incompletely assembled lipoproteins may be selectively retained and degraded. TG, triglycerides; Chol, cholesterol; CE, cholesterol esters; PL, phospholipids; RE, retinyl esters.
Related references:
- Luchoomun, J., and Hussain, M. M. (1999) Assembly and secretion of chylomicrons by differentiated Caco-2 cells: nascent triglycerides and preformed preformed phospholipids are preferentially used for lipoprotein assembly. J. Biol. Chem. 274:19565-19572.
- Nayak, N., Harrison, E.H., and Hussain, M. M. (2001) Retinyl ester secretion by the intestinal cells is a highly specific and regulated process that is dependent on the assembly and secretion of chylomicrons. J. Lipid Res. 42: 272-280.
- Hussain, M. M. (2000) A proposed model for the assembly of chylomicrons. Atherosclerosis. 148:1-15.
- Hussain, M. M., Kedees, M. H., Singh, K., Athar, H., Jamali, N. Z. (2001) Signposts in the assembly of chylomicrons. Front. Biosci. 6:D320-D331.
- Harrison, E. H., and Hussain, M. M. (2001) Mechanisms involved in the intestinal digestion and absorption of dietary vitamin A. J. Nutrition. 131:1405-1408.
2. PROTEIN-PROTEIN INTERACTIONS BETWEEN apoB AND MTP
Second, we are trying to understand how specific molecular interactions between apolipoprotein B (apoB) and microsomal triglyceride transfer protein (MTP) contribute to lipoprotein biosynthesis using various cellular, molecular and biochemical approaches. Lipoprotein biosynthesis is defective in abetalipoproteinemia due to mutations in the MTP gene whereas, in hypobetalipoproteinemia, plasma lipoprotein levels are low due to mutations in the apoB gene. These genetic disorders clearly underscore the importance of these two proteins in lipoprotein biogenesis and recent findings suggest that MTP and apoB interact during this process. We have demonstrated that MTP binds to apoB with high affinity, and that lysine and arginine residues in amino acids 270-570 of apoB are critical for its binding to MTP (8). At present, attempts are underway to identify amino acids critical for these protein-protein interactions. In addition, we have identified a novel antagonist, AGI-S17, which inhibits apoB-MTP interactions without affecting the lipid transfer activity of the MTP (9). Incubation of cells with this inhibitor results in decreased secretion of apoB polypeptides in liver-derived HepG2 and McA-RH7777 cells. Using AGI-S17 and site-directed mutagenesis, we plan to provide evidence that MTP acts as a chaperone for the assembly of apoB-containing lipoproteins. These studies are expected to provide novel targets for therapeutic interventions to decrease lipoprotein assembly and secretion.
Figure 2
Possible roles of apoB-MTP binding in lipoprotein assembly.
(A) Prevention of apoB degradation: (i) nascent apoB is depicted interacting with the inner leaflet of the endoplasmic reticulum (ER). MTP consists of "M" and "P" subunit. The M subunit contains independent apoB and lipid binding sites. MTP is shown to interact with nascent apoB. This binding may facilitate the translocation, lipidation and prevention of degradation of nascent apoB. (ii) If the apoB-MTP binding is inhibited by the use of inhibitors or mutations in the binding site, then apoB is predicted to undergo degradation involving hsp70 binding, ubiquitination, and proteosomal degradation.
Lipidation of nascent apoB: Lipidation of apoB can occur by two different pathways: (i) Free MTP that is not associated with lipid droplets or vesicles may bind nascent apoB. Subsequently, the apoB bound MTP can extract lipid molecules from the membrane and transfer them to nascent apoB polypeptide. Note that this process may involve formation of a pocket. Several rounds of this process will result in extensive lipidation of apoB. MTP molecules that are not physically associated with apoB can further assist this step. (ii) MTP associated with lipid vesicles or droplets may bind to apoB and provide a lipid core for the nascent apoB to encircle and wrap around it. This would result in an efficient and faster assembly of apoB-containing lipoproteins and may predominate during excess availability of fat. Release of MTP would result in the formation of secretion-competent primordial lipoproteins. Primordial lipoproteins can be supplied with a large dose of fat resulting in their "core expansion" and formation of "nascent lipoproteins". Droplets containing MTP molecules can efficiently accomplish the process of "core expansion" by fusing with primordial lipoproteins. However, experimental data for such a process is not yet available.
Pertinent References:
- Hussain, M. M., Bakillah, A., and Jamil, H. (1997) Apolipoprotein B binding to microsomal triglyceride transfer protein decreases with increases in length and lipidation: implications in lipoprotein biosynthesis. Biochemistry. 36:13060-13067.
- Bakillah, A., Jamil, H., and Hussain, M. M. (1998) Lysine and arginine residues in the N-terminal 18% of apolipoprotein B are critical for its binding to microsomal triglyceride transfer protein. Biochemistry. 37:3727-3734.
- Hussain, M. M., Bakillah, A., Nayak, N., and Shelness, G. S. (1998) Amino acids 430-570 in apolipoprotein B are critical for its binding to microsomal triglyceride transfer protein. J. Biol. Chem. 273:25612-25615.
- Bakillah, A., Nayak, N., Saxena, U., Medford, R. M. and Hussain, M. M. (2000) Decreased secretion of apoB follows inhibition of apoB-MTP binding by a novel antagonist. Biochemistry. 39:4892-4899.
- Bakillah, A., and Hussain, M. M. (2001) Binding of microsomal triglyceride transfer protein to lipids results in increased affinity for apolipoprotein B: Evidence for stable microsomal MTP/lipid complexes. J. Biol. Chem. 276:31466-31473. http://www.jbc.org/cgi/reprint/276/33/31466.pdf
Jahangir Iqbal, PhD, Postdoctoral Fellow
Paul Rava, M.D., PhD Student
Kamran Anwar, PhD Student
Kezhi Dai, PhD Student
Angelina Daysudova, BS, Research Student
Zeng-Xiu Liu, MD Research Associate
Robert Cornwall, Technician
Honors and Awards
1998: Irvine H. Page Award, Council on Arteriosclerosis, Thrombosis, and Vascular Biology, American Heart Association, National Center
1998: Leonard N. Horowitz Research Award, American Heart Association, Southeastern Pennsylvania Affiliate
1999-2002: Established Investigator, American Heart Association, National Center
2000: Director, Downstate Lipid and Vascular Biology Club
1999-2001: President, New York Lipid and Vascular Biology Club, Rockefeller University, New York, NY
2001: nternational Journal of Oncology, Oncology Reports, International Journal of Molecular Medicine Award For Outstanding Achievement, Crete, Greece
1995-1997: American Heart Association, Southeastern Pennsylvania Affiliate
1996: Ad hoc reviewer of grants for the Veterans Health Administration
1999: Special Study Section for Program Project, ZRG1 SSS-2, NIH, April 6-7.
1999: General Medicine A-2 Study Section, National Institutes of Health, Feb. 22-23
2000: SSS-3 Study Section Review Panel, National Institutes of Health
2000: WHO Regional Office for Europe, Copenhagen, Denmark
2001: Northeast Consortia Peer Review Study Group II, American Heart Association
2001: SSS-3 (20L) Postdoctoral (NRSA) Application Review Panel, NIH, Feb. 20-21
2001: ZRG1 SSS-T 01, Special Emphasis Panel, NIH, June 18, 2001
2001: Fonds zur Förderung der wissenschaftlichen Forschung, Austrian Science Fund,
Austria
Journal of Biological Chemistry
European Journal of Clinical Investigation
Journal of Lipid Research
Arteriosclerosis, Thrombosis, and Vascular Biology
Biochimica Biophysica Acta; Lipids
Journal of Clinical Investigation
American Journal of Physiology
Proceedings of the National Academy of Sciences, USA
FASEB J. American Journal of Cardiology, Biochemical Journal.
Managing Editor: Frontiers in Biosciences
Guest Editor: Cardiovascular Drug Reviews
1998: Co-Chair, Tenth Mid-Atlantic Lipid Research Symposium, March 4-6, Atlantic, City, NJ.
2001:Co-Chair, Thirteenth Mid-Atlantic Lipid Research Symposium, March 1-2, Atlantic City, NJ.
2001: Co-Chair, Lipid Seminar and Preceptorship, Cardiovascular Division, Downstate Medical Center, Brooklyn, NY
- Bakillah, A., Nayak, N., Saxena, U., Medford, R. M. and Hussain, M. M. (2000) Decreased secretion of apoB follows inhibition of apoB-MTP binding by a novel antagonist. Biochemistry. 39:4892-4899.
- Hussain, M. M., Obunike, J. C., Shaheen, A., Hussain, M. J., Shelness, G. S. and Goldberg, I. J. (2000) High affinity binding between lipoprotein lipase and lipoproteins involves multiple ionic and hydrophobic interactions, does not require enzyme activity, and is modulated by glycosaminoglycans. J. Biol. Chem. 275: 29324-29330.
- Nayak, N., Harrison, E.H., and Hussain, M. M. (2001) Retinyl ester secretion by the intestinal cells is a highly specific and regulated process that is dependent on the assembly and secretion of chylomicrons. J. Lipid Res. 42: 272-280.
- Bakillah, A., and Hussain, M. M. (2001) Binding of microsomal triglyceride transfer protein to lipids results in increased affinity for apolipoprotein B: Evidence for stable microsomal MTP/lipid complexes. J. Biol. Chem. 276:31466-31473. http://www.jbc.org/cgi/reprint/276/33/31466.pdf
- Babinska, A., Kedees, M. H., Athar, H., Sobocki, T., Sobocka, M. B., Ahmed, T., Ehrlich, Y. H., Hussain, M. M., and Kornecki, E. (2002) Two regions of the human platelet receptor (F11R) are critical for platelet aggregation, potentiation, and adhesion. Thromb. Haemost. 87:712-721.
- Kumar, M. S., Carson, M., Hussain, M. M., Murthy, H. M. K. (2002) Structures of apolipoprotein A-II and a lipid-surrogate complex provide insights into apolipoprotein-lipid interactions. Biochemistry 41:11681-11691.
- During, A., Hussain, M. M., Morel, D. W., and Harrison, E. H. (2002) Carotenoid uptake and secretion by Caco-2 cells: _-carotene isomer selectivity and carotenoids interactions. J. Lipid Res. 43:1086-1095.
- Babinska, A., Kedees, M. H., Athar, H., Ahmed, T., Batuman, O., Ehrlich, Y. H., Hussain, M. M., and Kornecki, E. (2002) F11-Receptor (F11R/JAM) mediates platelet adhesion to endothelial cells: role in inflammatory thrombosis. Thromb. Haemost. 88:843-850.
- Singh, K., Batuman, O. A., Akman, H., O., Kedees, M. H., Vakil, V., and Hussain, M. M. (2002) Differential, tissue-specific, transcriptional regulation of apolipoprotein B secretion by transforming growth factor _. J. Biol. Chem. 277:39515-39524.
- Kummrow, E., Hussain, M. M., Pan, M., Marsh, J.B., and Fisher, E.A. (2002) Myristic acid increases dense lipoprotein secretion by inhibiting apoB degradation triglycerides recruitment. J. Lipid Res. 43: 2155-2163.
List of Publications (Pub Med)
Recent ReviewsHussain, M. M. Strickland, D. K., Bakillah, A. (1999) The mammalian low-density lipoprotein receptor family. Ann. Rev. Nutr. 19:141-172. (One of the most cited review of the journal, ranked 8 out of the 50 most cited articles as of 10-01-2001)
Hussain, M. M. (2000) A proposed model for the assembly of chylomicrons. Atherosclerosis. 148:1-15.
Hussain, M. M., Kedees, M. H., Singh, K., Athar, H., Jamali, N. Z. (2001) Signposts in the assembly of chylomicrons. Front. Biosci. 6:D320-D331.
Hussain, M. M. (2001) Structural, biochemical and signaling properties of the low-density lipoprotein receptor gene family. Front. Biosci. 6:D417-D428.
Harrison, E. H., and Hussain, M. M. (2001) Mechanisms involved in the intestinal digestion and absorption of dietary vitamin A. J. Nutrition. 131:1405-1408.
Iqbal, J., Yakubov, R., and Hussain, M. M. (2003) Abetalipoproteinemia. In "The NORD Guide to Rare Diseases". Medical Editor: Edward Gruson. Publishers: Lippincott, Williams, & Wilkins. Philadelphia, PA. Chapter 9:1. Page 358.
Yakubov, R., Iqbal, J., and Hussain, M. M. (2003) Chylomicron Retention Disease. In "The NORD Guide to Rare Diseases". Medical Editor: Edward Gruson. Publishers: Lippincott, Williams, & Wilkins. Philadelphia, PA. Chapter 9:23. Page 380.
Hussain, M. M., Shin, J., Dreizen, P. (2003) Microsomal triglyceride transfer protein and its role in apoB-lipoprotein assembly. J. Lipid Res. 44:22-32.